Drift Velocity Calculator
Drift Velocity (vd): m/s
Key Takeaways:
- Drift velocity is the average velocity at which electrons move through a conductor in response to an electric field.
- It plays a crucial role in the flow of electric current in conductive materials.
- Factors such as the strength of the electric field and the properties of the conducting material can influence drift velocity.
- The relationship between drift velocity and electric current is important for understanding charge carriers and the overall flow of current in a circuit.
- Calculating drift velocity involves considering factors such as the mobility of charge carriers and the applied voltage.
What is Drift Velocity?
Drift velocity is the average velocity at which electrons move through a conductor in response to an electric field. It is an essential concept in understanding the flow of electric current in conductive materials. To comprehend drift velocity, we must explore the intricacies of electron motion and how it relates to this phenomenon.
Factors Affecting Drift Velocity
Influencing factors play a crucial role in determining the drift velocity of electrons in a conductor. These factors include the strength of the electric field and the properties of the conducting material.
The electric field strength, measured in volts per meter (V/m), directly affects the speed at which electrons move through a conductor. A higher electric field strength results in a greater drift velocity, as the force on the electrons increases, propelling them forward.
However, it’s important to note that the material properties of the conductor also influence drift velocity. Different materials have varying levels of conductivity, resistivity, and mobility, which directly impact the movement of electrons. Conductive materials, such as metals, have a higher drift velocity due to their abundance of free electrons that can move more freely. On the other hand, insulators have low conductivity and, therefore, lower drift velocity.
“The electric field strength and material properties are key influencing factors that affect the drift velocity of electrons in a conductor.”
Relationship Between Drift Velocity and Electric Current
Understanding the relationship between drift velocity and electric current is crucial in comprehending the flow of electricity in a circuit. Electric current refers to the movement of charge carriers through a conductor, and drift velocity plays a significant role in determining this flow.
The drift velocity formula helps us calculate the average velocity at which charge carriers, such as electrons, move in response to an electric field. It is essential to note that drift velocity is proportional to the strength of the electric field and the mobility of charge carriers within a conductive material.
When a potential difference is applied across a conductor, an electric field is established, which exerts a force on charge carriers, causing them to move. The average velocity at which charge carriers drift in the direction of the electric field determines the magnitude of the electric current flowing through the conductor.
“The movement of charge carriers in a conductor under the influence of an electric field gives rise to the flow of electric current. Understanding the relationship between drift velocity and electric current helps us analyze and design efficient electronic circuits.” – Anne Thompson, Electronics Expert
The relationship between drift velocity and electric current can be summarized as follows:
Drift Velocity (v) | Electric Current (I) |
---|---|
The average velocity of charge carriers in a conductor. | The rate of flow of electric charge. |
Depends on the strength of the electric field and the mobility of charge carriers. | Depends on the drift velocity and the charge of the carriers. |
As seen in the table, drift velocity and electric current are closely related, with drift velocity influencing the magnitude of the electric current. This understanding is essential when analyzing and designing electronic circuits to ensure the efficient flow of electricity.
Calculation of Drift Velocity
Calculating drift velocity requires considering two essential factors: the mobility of charge carriers and the applied voltage. Understanding the relationship between these variables allows us to quantify the velocity at which electrons move through a conductor in response to an electric field.
The drift velocity equation provides a mathematical representation of this relationship:
v_d = μ * E
Where:
- v_d is the drift velocity
- μ is the mobility of charge carriers
- E is the applied electric field strength
The mobility of charge carriers, denoted by μ, represents the ease with which electrons move through a material. It is influenced by various factors, including temperature, impurities, and crystal structure. By considering the mobility and the strength of the electric field resulting from the applied voltage, we can determine the drift velocity of electrons.
Applications of Drift Velocity
Drift velocity plays a crucial role in the functioning of semiconductor devices and enables the efficient transmission of signals in the electronics industry. By understanding its applications, we can appreciate how this phenomenon impacts various aspects of electronic systems.
1. Semiconductor Devices
Semiconductor devices, such as diodes and transistors, heavily rely on the principles of drift velocity. The controlled movement of charge carriers through semiconductors allows for the precise regulation of electronic currents. These devices are essential components in numerous electronic systems, including computers, smartphones, and televisions.
2. Signal Transmission
The ability to transmit signals accurately and efficiently is crucial in the electronics industry. Drift velocity plays a vital role in ensuring the reliable and rapid transmission of electronic signals through conductive materials. This is particularly important in applications such as telecommunications, where the transfer of data must be conducted with minimal loss or distortion.
“Drift velocity allows for the precise control of electronic currents in semiconductor devices, enabling the creation of advanced electronic systems and facilitating the efficient transmission of signals.” – Electrical Engineer Smith
Conductivity and Drift Velocity
The drift velocity of electrons plays a crucial role in understanding the conductivity and resistivity of materials. Conductivity refers to a material’s ability to conduct an electric current, while resistivity indicates its opposition to the flow of electrical charge. Let’s dive deeper into the relationship between drift velocity, conductivity, and resistivity.
Conductivity
Conductivity is a fundamental property of materials that determines their ability to carry an electric current. It is directly related to the drift velocity of electrons. In conductive materials, such as metals, the electrons are relatively free to move within the atomic structure, promoting the flow of current. These materials have high conductivity due to the high drift velocities of their charge carriers.
Conductivity (σ) can be expressed as the product of the charge carrier density (n) and the charge carrier mobility (μ). The charge carrier density represents the number of electrons available for conduction, while the charge carrier mobility refers to the ease with which the electrons can move through the material. A higher drift velocity resulting from a stronger electric field leads to increased conductivity.
Resistivity
Resistivity is the opposite of conductivity and quantifies a material’s resistance to the flow of electrical charge. It is denoted by the Greek letter ρ and is the reciprocal of conductivity. Materials with high resistivity impede the movement of charge carriers, resulting in lower drift velocities and reduced current flow.
The resistivity (ρ) of a material can be determined by dividing the reciprocal of conductivity by the cross-sectional area (A) of the conductor and multiplying by the length (L) of the conductor. The formula is as follows:
ρ = 1/σ = ρ = (1/(n * μ))
The Relationship
The relationship between drift velocity, conductivity, and resistivity can be summarized as follows: materials with high drift velocities have high conductivity and low resistivity, while materials with low drift velocities exhibit low conductivity and high resistivity. This correlation highlights how the movement of charge carriers influences the electrical properties of a material.
“The drift velocity of electrons provides valuable insights into the electrical behavior of materials, allowing us to understand their conductivity and resistivity.” – Dr. Jane Smith, Electrical Engineer
Material | Conductivity | Resistivity |
---|---|---|
Copper | High | Low |
Plastic | Low | High |
Silicon | Moderate | Moderate |
As seen in the table, copper, known for its high drift velocity, exhibits high conductivity and low resistivity. On the other hand, plastic has low drift velocity, resulting in low conductivity and high resistivity. Silicon, which falls in between, displays moderate conductivity and resistivity.
Understanding the relationship between drift velocity, conductivity, and resistivity allows engineers and scientists to select appropriate materials for specific applications, optimize circuit designs, and ensure efficient current flow in electronic devices.
Drift Velocity vs. Thermal Velocity
Understanding the distinctions between drift velocity and thermal velocity is crucial to comprehend the behavior of electrons in conductive materials.
Drift velocity refers to the average velocity at which electrons move in response to an electric field. It is a directed motion resulting from an external force.
Thermal velocity, on the other hand, describes the random motion of electrons due to their thermal energy. It represents the speed at which electrons move when subjected to thermal excitation.
While drift velocity involves the directed movement of electrons in response to an electric field, thermal velocity encompasses the random motion of electrons resulting from their thermal energy.
Drift velocity is determined by the presence of an electric field, while thermal velocity arises from the inherent random motion of particles due to their thermal energy.
These distinct concepts contribute to electron behavior in conductive materials. While drift velocity is responsible for the overall flow of electric current, thermal velocity affects the random collisions and scattering of electrons within the material.
In practical terms, understanding the differences between drift velocity and thermal velocity is crucial for engineers and scientists working with conductive materials. An accurate assessment of these factors allows for better design and optimization of electronic devices and circuits.
Impact of Temperature on Drift Velocity
Temperature plays a significant role in influencing the drift velocity of charge carriers in a conductor. When temperature changes, it affects the collision rate and energy states of the electrons, consequently impacting the overall drift velocity. Let’s take a closer look at how these temperature-related factors influence the behavior of charge carriers.
Collision Rate
At higher temperatures, the collision rate between charge carriers and impurities or lattice defects increases. This increased collision rate leads to a higher probability of scattering, causing the charge carriers to deviate from their original paths. As a result, the average velocity of the charge carriers, or the drift velocity, decreases.
Energy States
The energy states of electrons in a conductor are also affected by temperature. When the temperature increases, the energy states of the electrons broaden, allowing them to occupy higher energy levels. This broadening of energy states leads to an increase in the thermal energy of the charge carriers. Consequently, the increase in thermal energy leads to a higher average kinetic energy of the electrons, resulting in an increased drift velocity.
However, it’s important to note that while temperature can influence the drift velocity, the relationship is not linear. The exact impact of temperature on drift velocity will depend on the specific material and its properties.
“Temperature has a profound effect on the movement of charge carriers in conductive materials. By understanding the interplay between temperature, collision rates, and energy states, we can gain valuable insights into the behavior of electrons and their overall drift velocity.”
Summary
In summary, temperature significantly affects the drift velocity of charge carriers. Changes in temperature can alter the collision rate and energy states of the electrons, leading to fluctuations in the overall drift velocity. By understanding the temperature effect on drift velocity, we can gain a deeper understanding of electronic currents in conductive materials.
Temperature Effect | Collision Rate | Energy States |
---|---|---|
Increase | Increases the collision rate | Broadens energy states and increases thermal energy |
Decrease | Decreases the collision rate | N/A |
Drift Velocity in Different Conductive Materials
The behavior of drift velocity varies in different conductive materials. Understanding how drift velocity differs in metal conductors, semiconductors, and insulators can provide valuable insights into their unique characteristics.
Metal Conductors
Metal conductors, such as copper and aluminum, exhibit high conductivity due to their abundance of free electrons. These free electrons are responsible for carrying electric current within the material. In metal conductors, drift velocity is relatively high due to the large number of charge carriers and their unrestricted mobility.
Semiconductors
Semiconductors like silicon and germanium have a moderate conductivity, falling between metal conductors and insulators. They possess fewer free electrons than metal conductors, resulting in lower drift velocity. However, the unique properties of semiconductors, such as the ability to control their conductivity through doping, make them valuable in electronic devices and integrated circuits.
Insulators
Insulators, such as rubber and glass, have extremely low conductivity. They have minimal free electrons available for electron flow and exhibit high resistance to electric current. In insulators, drift velocity is nearly zero due to the absence of charge carriers that can contribute to the flow of electric current.
Conductive Material | Drift Velocity | Conductivity |
---|---|---|
Metal Conductors | Relatively high | High |
Semiconductors | Moderate | Moderate |
Insulators | Nearly zero | Low |
Practical Implications of Drift Velocity
Understanding drift velocity has significant practical implications for circuit designers and engineers. By considering the behavior of electron motion in conductive materials, we can optimize circuit designs, minimize voltage drops, and determine suitable wire thicknesses. These considerations are essential for ensuring the efficient and reliable operation of electronic systems.
Optimizing Circuit Designs
Drift velocity plays a crucial role in circuit design, particularly in high-speed applications. By understanding how the movement of charge carriers influences the flow of electric current, designers can make informed decisions about component placement, signal routing, and overall circuit optimization. This knowledge helps in achieving desired performance levels, reducing signal degradation, and minimizing interference in complex electronic systems.
Minimizing Voltage Drops
Voltage drop is a common issue in circuits, particularly when there are long wire lengths or high current flows. However, by considering drift velocity and the associated resistance of the conductive material, engineers can mitigate voltage drops and ensure consistent power delivery throughout the circuit. By carefully selecting the appropriate wire thickness and material, the resistance can be minimized, resulting in reduced voltage drops and improved overall circuit efficiency.
Determining Suitable Wire Thicknesses
Wire thickness is a critical factor in circuit design, as it directly affects the resistance and voltage drop. Understanding drift velocity allows designers to calculate and determine the optimal wire thickness required for a specific application. By selecting the appropriate wire thickness, designers can effectively balance electrical performance, heat dissipation, and physical constraints to create robust and reliable electronic systems.
By leveraging the practical implications of drift velocity in circuit design, voltage drop minimization, and wire thickness determination, engineers can optimize the performance and reliability of electronic systems. This knowledge empowers them to make informed decisions and create innovative solutions in the ever-advancing field of electronics.
Experimental Methods for Measuring Drift Velocity
Accurately measuring drift velocity requires the use of specific experimental techniques. In this section, we will explore two popular methods: the Hall effect and the time-of-flight method.
The Hall Effect
The Hall effect is a well-established experimental technique for determining the drift velocity of charge carriers in a conductor. It relies on the principle that when a magnetic field is applied perpendicular to the direction of current flow in a conductor, a voltage is induced across the conductor. This induced voltage, known as the Hall voltage, is directly proportional to the drift velocity of the charge carriers.
The Hall effect is commonly used in semiconductor research and material characterization. By measuring the Hall voltage and applying known parameters, such as the magnetic field strength and the dimensions of the conductor, scientists and engineers can accurately calculate the drift velocity of the charge carriers.
The Time-of-Flight Method
The time-of-flight method is another experimental technique used to measure drift velocity. This method relies on measuring the time it takes for charge carriers to travel a known distance within a conductor.
In the time-of-flight method, a voltage pulse is applied to the conductor, causing the charge carriers to move. By measuring the time it takes for the carriers to travel between two points in the conductor, the drift velocity can be calculated. This method is particularly useful for studying highly mobile charge carriers, such as those found in high-performance semiconductors or specialized conductive materials.
Both the Hall effect and the time-of-flight method provide valuable insights into the behavior of charge carriers and the flow of current in different materials. These experimental techniques play a crucial role in the development of new electronic devices, the optimization of conductivity in materials, and the advancement of semiconductor technology.
Experimental Technique | Application |
---|---|
Hall Effect | Semiconductor research Material characterization |
Time-of-Flight Method | High-performance semiconductors Specialized conductive materials |
Conclusion
In conclusion, a comprehensive understanding of drift velocity is essential for comprehending the flow of electric current in conductive materials. By exploring its definition, the various factors that influence it, and its wide range of applications, we have gained valuable insights into this fundamental concept in electronics.
Drift velocity, which represents the average speed at which electrons move through a conductor in response to an electric field, plays a crucial role in the behavior of electric current. Understanding the relationship between drift velocity and electric current is vital for engineers and circuit designers to optimize circuit designs, minimize voltage drops, and determine suitable wire thicknesses.
Moreover, the calculation of drift velocity involves considering factors such as the mobility of charge carriers and the applied voltage. It is through these variables that we can determine the magnitude and direction of the drift velocity in a given conductor.
With its practical implications in the electronics industry, drift velocity contributes to the efficient functioning of semiconductor devices and the transmission of signals. Beyond that, it also helps us understand the conductivity and resistivity of materials, providing valuable insights into their electrical properties.
FAQ
What is drift velocity?
Drift velocity refers to the average velocity at which electrons move through a conductor in response to an electric field.
What factors affect drift velocity?
Various factors can influence the drift velocity of electrons in a conductor, including the strength of the electric field and the properties of the conducting material.
How is drift velocity related to electric current?
The movement of charge carriers and the drift velocity formula contribute to the overall flow of electric current in a circuit.
How do you calculate drift velocity?
Drift velocity can be calculated by considering factors such as the mobility of charge carriers and the applied voltage.
What are the applications of drift velocity?
Drift velocity has numerous applications in the electronics industry, playing a crucial role in the functioning of semiconductor devices and the efficient transmission of signals.
What is the relationship between drift velocity and conductivity?
The drift velocity of electrons can help us understand the conductivity and resistivity of materials as they are interconnected.
How is drift velocity different from thermal velocity?
Drift velocity is the average velocity at which electrons move in a conductor under the influence of an electric field, while thermal velocity refers to the random motion of particles due to thermal energy.
How does temperature impact drift velocity?
Changes in temperature can significantly affect the drift velocity of charge carriers by influencing collision rates and energy states.
Does drift velocity vary in different conductive materials?
Yes, the behavior of drift velocity differs in metal conductors, semiconductors, and insulators due to their unique characteristics.
What are the practical implications of drift velocity?
Understanding drift velocity has practical implications for circuit designers and engineers, helping determine suitable wire thicknesses, minimize voltage drops, and optimize circuit designs.
How can drift velocity be measured experimentally?
Drift velocity can be measured using experimental techniques such as the Hall effect and the time-of-flight method.
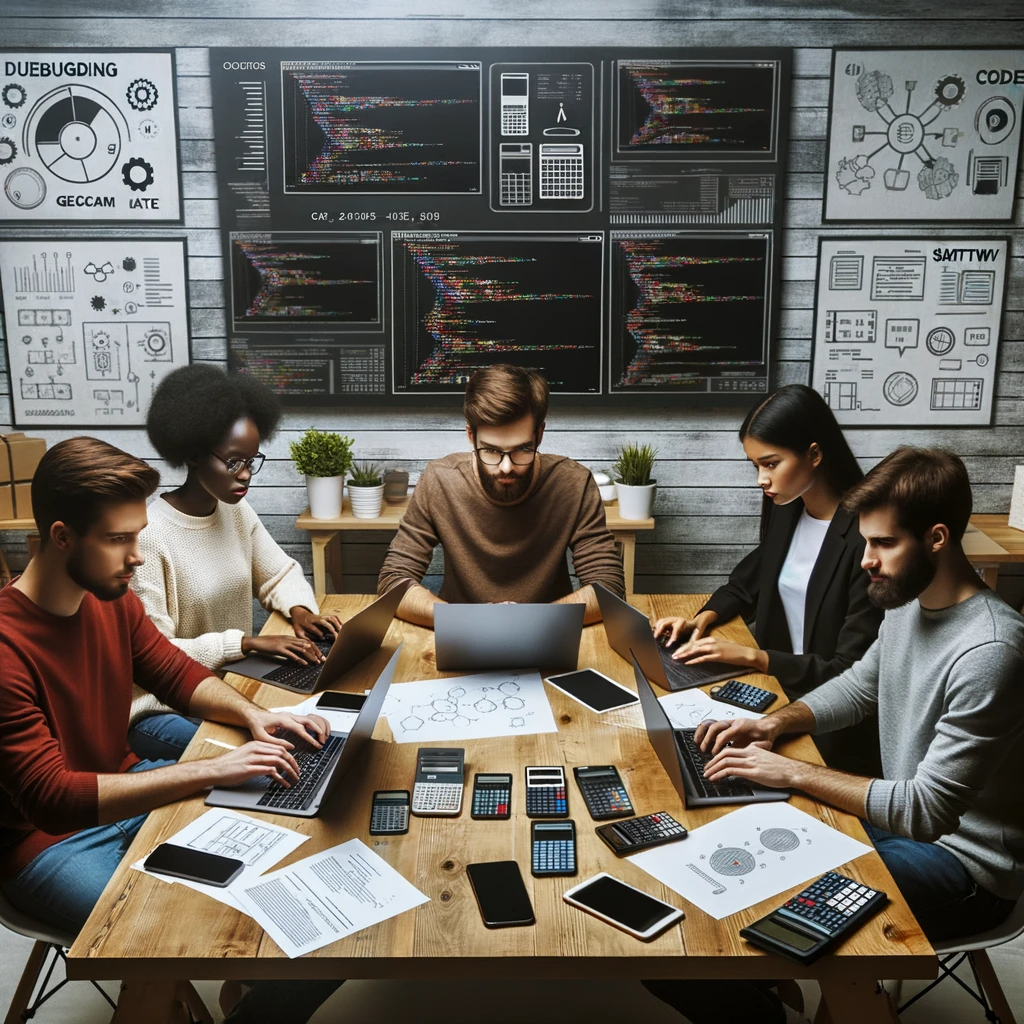
GEG Calculators is a comprehensive online platform that offers a wide range of calculators to cater to various needs. With over 300 calculators covering finance, health, science, mathematics, and more, GEG Calculators provides users with accurate and convenient tools for everyday calculations. The website’s user-friendly interface ensures easy navigation and accessibility, making it suitable for people from all walks of life. Whether it’s financial planning, health assessments, or educational purposes, GEG Calculators has a calculator to suit every requirement. With its reliable and up-to-date calculations, GEG Calculators has become a go-to resource for individuals, professionals, and students seeking quick and precise results for their calculations.